
Atin PAL's Group
Quantum Transport and Device Lab
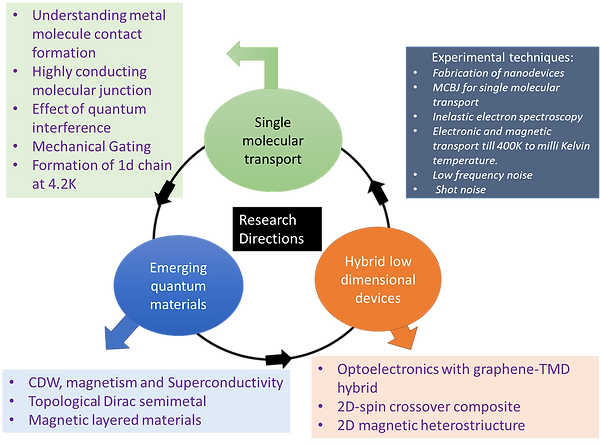
Transport through atomic and single molecular junction
A single organic molecule suspended between two metallic electrodes is an attractive electronic device since it allows using the rich structural possibilities of organic molecules to manipulate electronic conductance at the nano-scale. Up to date, most of the research concerning single-molecule conductance, focused on systems where the molecule is attached to the metal electrodes (mostly with gold) via anchoring groups, which act as a potential barrier and suppress the conductance to the tunneling regime. Recent experiments reveal that metal-molecule hybridization plays a key role in determining the electronic properties of a molecular junction. In this project, we intend to study both electric and thermoelectric properties of single molecular junction by changing the functionality of both the electrode and molecule by mechanically controllable break junction technique. Both s-metal (Au, Ag, Cu etc.) and d-metal (Pt, Pd, Fe, Ni etc.) will be used as electrodes, whereas, different molecular functionalities will be explored. Here we intend to identify the effect of the asymmetry of the molecule and the presence of a dipole moment along the molecule on the electronic transport properties of this highly conductive molecular junction. We will compare the conductance of two isomeric molecules, the symmetric and asymmetric molecules by attaching the molecules directly to metallic electrodes with no anchoring side groups. Understanding the dependence between structure and conductance will help us to learn how to control conductance in the atomic scale.
Hybrid low dimensional devices
​
​
The isolation of graphene by a simple scotch-tape based technique has created a huge playground for exploring various two-dimensional (2d) layered materials due to their excellent electronic, optical, mechanical and thermal properties. After graphene, lot of new 2D materials were discovered including insulators, semiconductors, superconductors, topological insulator, topological semimetals and two dimensional magnets. Superlattices and heterostructures have already been widely explored to tailor the electronic properties of two-dimensional electron systems. With today’s nanofabrication scheme it is possible align different 2D materials almost perfectly on top of each other to form heterostructures. Proximity effect is being emerged as a radically different path to transform a given material through its adjacent regions to become superconducting, magnetic, or topologically nontrivial. Such proximity effects not only is a ubiquitous approach compared to the conventional methods of doping or functionalization but also can overcome their various limitations. Our current research focusses on the following:
-
Fabricating high quality two-dimensional devices (graphene, transition metal dichalcognides (TMDCs) etc.) in combination with various functional molecules. Our immediate attention is to create hybrid devices in combination with spin crossover molecules and understand the transport mechanism.
-
Optoelectronics with TMDC based hybrids. In particular, we intend to create large area broadband photodetector using 2D-0D hybrids (e.g., CVD graphene with MoS2 or WS2 quantum dots).
-
We intend to explore spintronics and other emerging phenomena with 2d magnetic heterostructure in future.
​
Emerging quantum materials: magnetic, topological and superconducting materials
Our aim is to investigate some of the key issues in solid-state physics and materials science by studying new topological materials, semimetals, unconventional superconductivity, charge density wave physics. etc. in various emerging materials. We have established expertise in our group to investigate the electronic and magnetic properties of these materials (preferably single crystals) using electrical and magneto transport and magnetization measurements with our existing low temperature facilities at SNBNCBS.